Global Warmings Effect on Soil Carbon Microbial DecompositionTABLE OF CONTENTS Page NumberSUMMARY 1-2KEYWORDS (climate change, carbon cycling, Arrhenius equation, organic decomposition, thermal adaptation, microbial soil respiration)INTRODUCTION 2-3SOIL-CARBON DECOMPOSTION 3-5 Factors of organic decomposition 3-4 Formulas involved in decomposition rates 4-5PERMAFROST 5-8 Subarctic soil microbial biomass 6-7 Carbon exchange and release from thawing permafrost 7-8MICROBIAL SOIL RESPIRATION 8-11 Thermal adaptation 9-10 Environmental soil classes 10-11CONCLUSION 11-13REFERENCES 13[Cite your source here.]Summary In theory if the base formulas of microbial decomposition were the only elements at play, climate change and microbial decomposition would be involved in a global positive feedback loop. With climate change increasing temperature, decomposition rates will also increase and in turn provide additional CO2 that will perpetuate climate change. Terrestrial organic carbon being such a large portion of the global carbon supply, any increase in current global decomposition rates could have a profound effect. There are many other environmental components involved that prevent this from being quite as large of a positive feedback loop as theoretically possible.IntroductionClimate change has been a topic on peoples tongues for years. From the factors affecting climate change, to the effects climate change has on the Earth. There is an incredible amount of research being done on the subject. Aboveground climate change effects have become more well known, while underground parts of the ecosystem have been neglected. The main effect terrestrial organisms can have on climate change is through carbon cycling. Soil flora reduce atmospheric carbon, while root respiration and microbe aided decomposition adds to atmospheric carbon (1).The processes involved in plants participation of organic carbon cycling is fairly well understood. Soil-carbon decomposition aided by microbes has many factors that are starting to be researched more heavily. A core aspect of decomposition that is uniquely interesting to climate change is its sensitivity to increases in temperature (1,2). In theory if the rate of decomposition increases with increases in temperature; then a positive feedback loop may occur as the CO2 produced through decomposition will help raise earths temperature, in turn raising decomposition rates (3). Studies have shown however that this increased rate of carbon release into the atmosphere reduces back to normal levels after several years (4). Measuring the effect different processes have on the climate is already difficult due to the duration it takes to see real changes. As we learn more about decompositions place in this process, more questions get raised at the same time.Soil-Carbon Decomposition In the global organic carbon supply, a large percentage of it exists in the worlds wetlands, peatlands, permafrost, and upland soil (1). There is a significant amount more organic carbon in the worlds soil than in the worlds atmosphere. Soil contains several thousand various organic carbon compounds in a variety of combinations (1). Different types of soil contain various amounts of the global carbon as seen in Table 1 (1). These carbons compounds decompose as part of the global carbon cycle, thus playing a part in climate change. Due to differences in soil conditions upland soil has more involved decomposition; while wetlands, peatlands, and permafrost have lower rates of decomposition leading to higher carbon densities (1). Warming temperatures can lead to increases in decomposition rates, affecting permafrost and peatlands due to their higher latitudes (1). There are many factors at play with decomposition rates.Factors of organic decomposition Decomposition of organic carbon is a complex process involving many different factors. Much of the carbon in the decomposition cycle is imputed by leaf and root detritus, during decomposition CO2 and CH4 is outputted (1). While root respiration makes much more CO2, microbial decomposition of organic matter is a temperature dependent process that produces a large amount of global CO2 (1,3,4). At the core of microbial decomposition of organic carbon is the microbes ability to get past the activation energy needed to decompose the various organic carbon molecules. The process is temperature dependent as an increase in temperature provides more energy needed to get past the activation energy. The activation energy is related to the molecular structure of the organic carbon and its ambient temperature (1). Decomposition is temperature sensitive, as molecular complexity of organic carbon increases the temperature sensitivity of decomposition will also increase (1). Activation energy becomes greater as molecular complexity increases, making the process of decomposition more sensitive to temperature changes (1).Formulas involved in decomposition rates-Davidson There are several formulas involved in measuring the rate changes of biological systems. With decomposition being temperature sensitive, the Arrhenius equation is used to find the relative reaction rate. The Arrhenius equation says changes in relative reaction rates is a function of temperature (1). Relative rate of decomposition of soil organic matter with high activation energy might be sensitive to temperature, but changes in absolute rate will most likely be hard to detect (1). The basis of the Arrhenius equation was used to form the formula for Q10 to find the absolute rate.k=Ae(-Ea/RT)Arrhenius equationk is the reaction rate constantA is the frequency factor (theoretical reaction rate constant in the absence of activation energy)Ea is the required activation energyR is the gas constant (8.314 J K-1mol-1)T is temperature in kelvinsQ10 is the temperature coefficientR is the rateT is the temperature in kelvins Q10 is a necessary factor to use when evaluating rate changes. Q10 is the factor that reaction rates increase with a 10° rise in temperature. For most systems the Q10 is 2-3, and according to Davidsons article A rule of thumb widely accepted in the biological research community is that the rate of decomposition of SOM, like any other biological reaction rate, tends to double for every 10o rise in temperature (1). According to the Arrhenius equation, the Q10 should decrease as the temperature increases, which is shown in the top half of Figure #. The theory for why this happens is the lack of molecules that need the higher temperature to react (1). There is a visible increase in the slant of the data in Figure 1, showing that as activation energy increases the sensitivity to temperature also increases.Permafrost There has been a marked trend over the past century of warming throughout artic regions (5). The Arctic Climate Impact Assessment (ACIA) projects 4-7°C temperature increase by year 2100 (5). This warming is projected to promote soil organic matter (SOM) decomposition. With permafrost containing a large concentration of organic carbon, this projected accelerated decomposition could have profound effects on the earth. Thawing permafrost shows most potential for positive climate feedback.Subarctic soil microbial biomass There was a long-term study done in a subarctic heath ecosystem of northern Sweden where they collected data for 15 years since 1989 (5). They manipulated the environment to simulate potential factors of climate change. Passive open-top greenhouses to simulate predicted warming, NPK fertilization to simulate increased nutrient availability, and shading cloths to simulate increased cloudiness (5). A variety of above and belowground reactions including plant species abundances, plant biomass, plant and soil nutrient content and C pools, microbial biomass, fluxes of CO2 and CH4 were studied (5). There was evidence that microbial biomass was more responsive to addition of carbon than fertilization, showing that carbon is potentially a limitation of microorganisms. As shown in Figure 2, there was statistically significant drop in soil carbon at depths 5 and 10 cm after being exposed to warming over 15 years. Showing evidence that as temperatures rise, so does the rate of decomposition. Shading also had a slight affect on decomposition, but much less statistically significant. The affect of warming wasnt notable until at least a decade had passed.As one could expect, fertilization had a large impact on the carbon quantity of the top soil, with some effect on the deeper soil. Adding fertilizer will obviously greatly increase the total nutrient amount if more nutrients than the microbes can physically process. This large increase was then counteracted by both warming and shading. With the increase of decomposition rates due to the warmer temperatures, a higher percentage of the added nutrients will be decomposed. On the top soil there was a larger decrease in carbon in the fertilized soil vs the control group. This may be due to nutrition being a limiting factor in the reaction. It can be inferred that the activation energy of the added carbon sources must be higher than the original carbon as the additional nutrients require warming to be decomposed. Both nutrient and temperature increases will increase the rate of decomposition. Although there was no data collected on CO2 production from decomposition, it can be assumed that with an increase in decomposition rates an increase in CO2 production will follow.Carbon exchange and release from thawing permafrost Permafrost soil is a large piece of relatively untouched organic carbon storage. The total carbon storage of the worlds permafrost is twice what the worlds atmosphere contains (6). To study the carbon exchange and release from thawing permafrost, a team measured an Alaskan tundra site over several years.Figure 3 is interesting in that it provides information that at first glance might appear to contradict previous theories. In reality it actually helps confirm the theory that global warming is increasing decomposition rates. Since decomposition increases with a temperature increase, one might be confused by the loss of atmospheric carbon during the summer months. But the greatly increased growth rates during the summer counteract the increased decomposition rates. While in the winter months there is near zero growth, but still warm enough for microbial reactions to occur. During warmer years, as identified by the dark bar showing an extensive thaw, there is larger amounts of atmospheric carbon intake. This trait is also evident during the early and late months of the growing season. Showing that it takes a much higher temperature for plants to begin growth, but warm enough for microbes to thrive. Microbes do especially well during the warmer years, plants however do better during the moderate years. This carbon exchange battle between plants and microbes shows the potential negative and positive feedbacks that will affect the earth.Microbial Soil Respiration Microorganisms decomposition of soil organic carbon produces atmospheric CO2 through respiration. The decomposition rates of soil organic carbon in the short term are dependent on temperature (4). If this temperature dependence was extended to the long term, the decomposition rates would keep increasing as world temperatures increased. Field experiments have shown that respiration rates enduring soil warming. return to normal after a few years (4). Two potential reasons for this rate return is substrate-depletion of carbon supply, or the microbes adapt to increased temperatures with thermal adaptation (4). There is obviously reason to believe that the carbon supply might be depleting faster than it can replenish itself, which would decrease the reaction rates. To properly test thermal adaptation without substrate-depletion being a factor, an excess of substrate can be added to the experiments.Thermal Adaptation The changes in respiration that occur due to temperature changes in microbes is defined by thermal adaptation. The term thermal adaptation could be used describe responses organisms show, from instantaneous temperature compensation to mutation selection. Bradford defines thermal adaptation as a decrease in heterotrophic soil respiration rates per unit microbial biomass in response to sustained increase in temperature (4). The same also applies to extended decreases in temperature. He normalizes for biomass because temperature change responses affect mass specific respiration rates (Rmass). Responses to instantaneous temperature changes dont affect Rmass,In Figure 4, three thermal adaptations of Rmass are graphically described. Type I adaptation shows soils adapted to higher temperatures, which exhibit a decrease in the Q10 of Rmass. In the heated soils here is no change in Rmass at low temperatures, and lower Rmass at intermediate and high temperatures. Type II adaptation has lower Rmass values at every temperature, with no change in Q10. Type III adaptation depicts cold adapted microbes being heated and thermal adaptation increases the optimal temperature for Rmass. It is possible for all of the above adaptations to occur at the same time in one soil microbial community (4).Environmental Soil Classes While most models for carbon decomposition use a fixed Q10 value, it is known to vary region to region. This is impart due to different environmental soil classes (ESCs). It is accepted that Q10 decreases as regions become closer to the equator; soil moisture also impacts soil respirations sensitivity to temperature. (2)In Figure 5 the Q10 was on average lowered by 5% when water holding capacity (WHC) was increased from 30% to 75%. There is also evidence that the soils pH value regulates the effect soil moisture has on Q10 (2). Q10 greatly increased with soil moisture when in high pH soil, while the opposite occurred to Q10 when in low pH soil (2). It can also be noted that soil moistures effect on Q10 is more noticeable when moving from very dry soil to intermediate soil moisture levels (2). This may be in part due to the near lack of moisture being a very limiting factor to decomposition. Although there is apparent variability within individual environmental soil classes, there is obvious trends between the different classes. This may be explained by pH or available organic carbon.Conclusion It is common knowledge that CO2 plays a large part in climate change. Organic carbon is constantly being moved between the atmosphere and the earth. A natural source of this atmospheric organic carbon comes from carbon cycling, and more specifically the decomposition of soil organic carbon step in carbon cycling. Carbon cycling is crucial aspect of climate change, in theory both can impact the other due to decompositions sensitivity to increased temperature and its production of CO2. Decomposition rates is proven to increase with increased temperatures, this is in part to increasing temperature being the simplest way to overcome the activation energy of more complex carbon compounds. Doing this increases the amount of organic carbon available for the reaction. Q10 is the value used to describe how much the rate increases with a temperature increase. Different sources of research have looked into the various factors that also have an effect on Q10 besides just temperature. One of the classic examples of how as the earth warms it increases the amount of organic carbon to cycle into the atmosphere is the earths permafrost. What makes permafrost unique from other warming regions is not only are complex carbons having their activation energy achieved, but due to the thawing of the soil simple carbons become available for decomposition. These simpler carbons, with low activation energies, tend to exist in larger quantities providing the process of decomposition with much more substrate per degree change; as compared to regions fighting for the energy to react with more complex organic carbon. Permafrosts carbon exchange operates at such low levels that its decomposition is in a constant tug of war with the plants removal of organic carbon from the atmosphere. At its core, decomposition rate is affected by temperature fluctuations. Several studies have shown that there are additional factors that impact decompositions sensitivity to temperature, including thermal adaptation and different environmental soil classes. Although decomposition rates increase with a temperature increase, field studies show that decomposition rates return to normal after several years of increased temperature. This may be due to substrate depletion and the thermal adaptation of the microbes. Studies showed that when substrate depletion is removed as a cause, decomposition rates still even out due to thermal adaptation. The Q10 and mass specific respiration rates are affected differently by temperature change depending on what temperature it is currently adapted to. The region in which the soil exists also impacts how Q10 changes with temperature changes. This is due to differences in soil organic carbon composition, soil moisture levels, and pH levels. Soil organic carbon composition is a simple factor as reaction rates increase with more available substrate. If there arent any carbons with activation energy within the temperature change range available for the reaction then an increased temperature wont increase the decomposition rate. Increasing soil moisture is shown to slightly decrease Q10; this effect is stronger when in a low pH, with the opposite happening in high pH soil. Microbial decomposition is shown to be a highly complicated process, that is part of an even more complicated global system. Although the reaction of decomposition is similar across the world, no region is affected similarly by the multitude of factors at play. There is a possibility that microbial decomposition could be involved in a massive global positive feedback loop. Much more research and long-term data accumulation must occur before a more concise answer can be given on that theory.ReferencesDavidson, E. A. and I. A. Janssens (2006). Temperature sensitivity of soil carbon decomposition and feedbacks to climate change. Nature 440: 165.Meyer, N., et al. (2018). The Temperature Sensitivity (Q10) of Soil Respiration: Controlling Factors and Spatial Prediction at Regional Scale Based on Environmental Soil Classes. Global Biogeochemical Cycles 32(2): 306-323.Allison, S. D., et al. (2010). Soil-carbon response to warming dependent on microbial physiology. Nature Geoscience 3: 336.Bradford, M. A., et al. (2008). Thermal adaptation of soil microbial respiration to elevated temperature. Ecology Letters 11(12): 1316-1327.Rinnan, R., et al. (2006). Fifteen years of climate change manipulations alter soil microbial communities in a subarctic heath ecosystem. Global Change Biology 13(1): 28-39.Schuur, E. A. G., et al. (2009). The effect of permafrost thaw on old carbon release and net carbon exchange from tundra. Nature 459: 556.

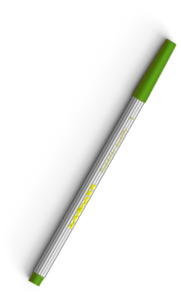

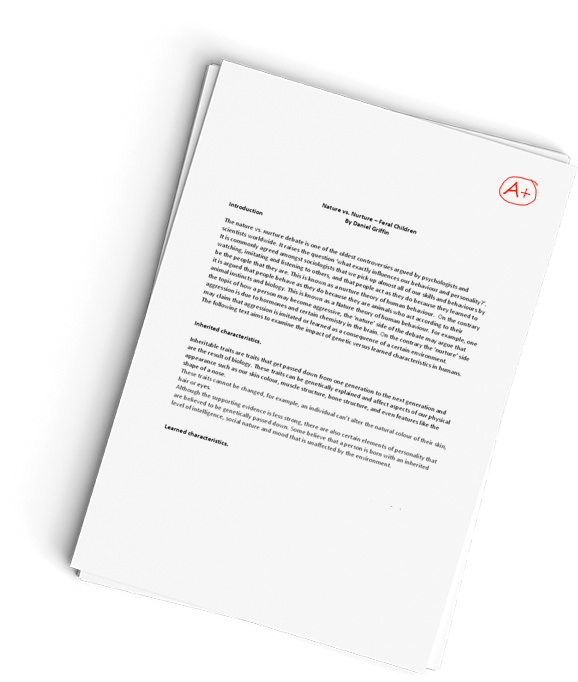

Global Warmings Effect on Soil Carbon Microbial Decomposition
Mar 17, 2020 | Geography
Recent Posts
- Develop a PowerPoint presentation on a clinical case for Anemia
- NURS6003N Module 6 Discussions
- WK 8: Ethics and the Role of IRB
- NURS 8310 Week 11 Final Paper Assignment : Major Assessment 7: Using an Epidemiological Approach to Critically Analyze a Population Health Problem
- NURS_8310 Week10 Assignment
Archives
- May 2024
- April 2024
- January 2024
- December 2023
- August 2023
- July 2023
- June 2023
- May 2023
- October 2022
- September 2022
- August 2022
- July 2022
- June 2022
- March 2022
- February 2022
- January 2022
- December 2021
- November 2021
- October 2021
- September 2021
- August 2021
- July 2021
- June 2021
- May 2021
- April 2021
- March 2021
- January 2021
- November 2020
- April 2020
- March 2020
- February 2020
- January 2020
- December 2019
- November 2019
- October 2019
- September 2019
Categories
- AN AUTOMATED EVALUATION OF LIVE FORENSIC APPROACH
- Saint Leo MGT 325 Finance for Managers Unit 8 – Term Paper/Project 2015Saint Le
- __________ occurs when the parties disagree on an issue and when its settleme
- —will only accept customized answers. Please assist with attached assignment.
- -the enemy are close upon them and among them
- -their ammunition must be nearly expended
- . Motorcade Company has three service departments (S1, S2, and S3) an
- .54541015625px;"="">.54541015625px;"="">.54541015625px;="" color:="" rgb(0,="" 0
- .baker.edu/webapps/blackboard/execute/uploadAssignment?content_id=_15643053_1&c
- .blackboard.com/webapps/blackboard/content/launchLink.jsp?course_id=_57864_1&con
- .blackboard.com/webapps/blackboard/content/launchLink.jsp?course_id=_60184_1&con
- .blackboard.com/webapps/blackboard/execute/uploadAssignment?content_id=_2459855_
- .d2l-table-0[dir=rtl] >thead~tbody:first-of-type>tr:first-child>td:last-child
- .equella.ecollege.com/file/93f10ff4-714a-4fe5-a1f8-faaffb47bfa5/43/MGMT404_CH_C
- .equella.ecollege.com/file/93f10ff4-714a-4fe5-a1f8-faaffb47bfa5/43/MGMT404_CH_Co
- .equella.ecollege.com/file/93f10ff4-714a-4fe5-a1f8-faaffb47bfa5/44/MGMT404_CH_Co
- .gif">.496000289917px;="" background-color:="" rgb(255,="" 255,="" 255);"="">Fi
- .gif">Kaplan University School of Business .gif">.gif"> GB533 Sales Fo
- .gif">Kaplan University School of Business .jpg"> GB533 Sales Force Ma
- .gif">Unit 8 [203: Human Resource Management] .gif"> Assignment Rubric .gif">
- .jpg" alt="KULogo">Assignment Template and Rubric Legal Aspects of HRM Assign
- .png" alt="Text box: purpose of this assignment this assignment demonstrates ho
- .umuc.edu/d2l/img/0/Shared.Main.actHide.png?v=10.3.0.791-144" alt="">Hide Folder
- .umuc.edu/d2l/img/lp/pixel.gif" alt="" 10.3.0.791-144)"="" -10px="" -1441px="" n
- .umuc.edu/d2l/img/lp/pixel.gif" alt="">Actions for Week 1 Discussion Topic 1Have
- .uul l illl'
- "" ""Qualitative Analysis Assignment: Transcript 3
- "" which is attached to this assignment.
- "Managing Change in Organizations" Please respond to the following:Determine why
- (1)Patient Satisfactory, (2) Worked Hours, (3) Liquidity, and (4) Profitability.
- (Boston: Houghton
- (Spring 2015 BMGT 1327 CRN 40722)Assignment:Chapter 2 Exam1.award:3 out of3.00 p
- (Spring 2015 BMGT 1327 CRN 40722)Assignment:Chapter 3 Exam1.award:3 out of3.00 p
- (Spring 2015 BMGT 1327 CRN 40722)Assignment:Chapter 4 Exam1.award:3 out of3.00 p
- (Supply Chain Logistics Management 444) To avoid material shortages and thereby
- (TCO 1) The vice president of marketing tells a marketing manager to prepare a
- (TCO 1) Which of the below is an accurate citation for a journal article
- (TCO 10). Consider this scenario: John Hopken is the new supervisor of a team
- [dir=rtl] .d2l-table-0 >*>tr>th:first-child
- { ""cell_type"": ""markdown""
- /files/0886109913504154-pdf,/files/1363460706065057-pdf,/files/0886109914560741-pdf
- /files/0e9b637b-7632-455d-a675-f5ea75dfd775-jpeg,/files/b6042c9d-cde8-48d8-acec-cc232a6adc48-jpeg
- /files/20191118150623part-vii-assessment-tool-development-rub1-pdf
- /files/20191119051422iom-report-future-of-nursing-rubric-xlsx
- /files/20200428035024defend-or-refute-guide-pdf
- /files/adjustmentdisorder-pdf,/files/depression1-pdf-6973733
- /files/assignment1j-docx-7815535,/files/assignment2j-docx
- /files/assignment2-docx-8830717
- /files/assignment3-3405029.docx
- /files/bookchap1-412-pdf,/files/bookchap1-412-pdf-7977187
- /files/businessculturaldimensionsanalysisgradingrubric-pdf-7789673"
- /files/chapter5-pdf-7822037
- /files/crjs3001wk1studentreplies-docx
- /files/cyberspacelawandrecommendations-doc"
- /files/developingobjectives1-docx,/files/article3assignments3-41-pdf,/files/developingobjectives1-docx-7919359
- /files/discussionreflectionguide-docx,/files/introduction-to-criminology-10th-edition-9781544339023-pdf,/files/sample-docx-7598097
- /files/essay2-documentedargumentrevised22-pdf-6947741
- /files/financialmanagementhw1-docx
- /files/frenchandindianwar-docx-7926377,/files/bostonteapartyaftermath-docx
- /files/homework2negotiationmorgan-docx,/files/homeworknegotiation1michele-docx
- /files/image24-jpeg-7528157,/files/image027-jpeg,/files/image115-jpeg
- /files/j01e2assignment41-docx
- /files/labassignmnetw8-docx-7665133
- /files/lessonweek4-docx
- /files/mat240houselistingpricebyregionpjct5-xlsx-7794861,/files/mat240modulefiveassignmenttemplatepjct5-docx-7794863
- /files/mathematicsfloridastandards-pdf-7190487"
- /files/microm53question-docx
- /files/milecture-pdf,/files/motivationalinterviewing-thebmj-pdf
- /files/nrnpprac6635comprehensivepsychiatricevaluationtemplate-docx-8263023"
- /files/outline-docx-6118175,/files/topic3-docx-6118177
- /files/principlesofcorporatefinance13erichardbrealey-pdf-7629311,/files/wk71-docx,/files/wk72-docx,/files/wk73-docx,/files/wk74-docx
- /files/problemstatementchecklist-pdf-6967979,/files/week4article-pdf-6967981
- /files/project-rubric21-docx
- /files/reflectionpaperguidelines-docx-7570139
- /files/rubricpandv-pdf-7584061,/files/page11-pdf-7584063,/files/page9-10-pdf-7584065,/files/implicationofpiagetsandvygotskytheories-summer2021socialstudiesmethodsforece-fieldece-3571-01-pdf
- /files/sec-516-t6-meetingtheneedsofdiverselearners-docx"
- /files/spd-400-d-t6-studentgoalsandinterventionplantemplate-docx-7938583,/files/rub-docx-7938623
- /files/supplychainmanagement-docx-7583157
- /files/taskdifficultyandincubation1-docx
- /files/topic-discussion1-andersondam-pdf
- /files/unitvinternationlstudyguide-pdf-7796117
- /files/week3assignmentg-docx
- /files/week4rubric-docx-7856191
- /files/week7readingdestructivebehavior-pdf-7890761,/files/bibliu-print-9781452236315table143-pdf
- /files/withgreatpower-examiningtherepresentationandempowermentof-pdf,/files/journal-vol-4-issue-1-3-pdf
- /files/woodch9-pdf,/files/chapter8-pdf-6111751
- /files/youthexposedtoviolenceindc-august2021-docx,/files/chapteronethenatureandtoolsofresearch-pdf
- & Doherty
- & Goldin-Meadow
- & Jacobs
- & Korn
- & Seefeldt C. (2014). Social Studies for the Preschool/Primary Child (Subscription). [VitalSource Bookshelf]. Retrieved from https://bookshelf.vitalsource.com/#/books/9780133560763/
- & Su
- & Wilberger
- ~I'vcrn I""theorems"" follow from it
- $1.00 Can you help me with Case and SLP Trident MGt301 full course 2014 latest
- 0
- 000 in income each year. Bill's income is about $25
- 000 to $200
- 000 to 700
- 0005
- 0007.102-Very Good05C$0$75
- 000Maximum Production 8
- 1 898).
- 1 out of 1 points Correct The process by which companies undertake some ac
- 1. (TCO 1) Which step of the project life cycle creates the project charter? (Po
- 1. An organizationâs human resources are a means by which the firm fosters a s
- 1. Companies must ALWAYS examine their pricing:based on the supply of the produc
- 1. Create a job description and specifications for your dream job.2. Design a co
- 1. Discuss the general procedure for determining the order quantity when price
- 1. Discuss the general procedure for determining the order quantity when price b
- 1. Fran, an executive with Global Sales Corporation, has to decide whether to ma
- 1. Human resource managers generally exert _____ within the human resources depa
- 1. Identify an important problem in your school, business, or organization that
- 1. Identify and describe at least three of the most difficult issues facing heal
- 1. Question : Costco has been successful in part due to its unique approach to s
- 1. The areas where management is more likely to misstate transactions are riskie
- 1. Two mutually exclusive investments cost $10,000 each and havethe following ca
- 1. What were two occupations O*NET found that fit your skills, interests, and ex
- 1. When customers are made to wait for a service, organizations can benefit fro
- 1.Reaching a valid decision is based on the evaluation of arguments. If we know
- 1) 10 points Located alongside a cobblestoned street in Old City, Old City Photo
- 1) Design is an important part of a well-delivered message. Give an example of a
- 1) Distrust, disrespect, and animosity pertain to which component of indirect
- 1) Distrust, disrespect, and animosity pertain to which component of indirect co
- 1) Which of the following is NOT true of the Internet Revolution as it relates t
- 1111 writing and reading is embedded in some Discourse
- 12 font
- 12 point font
- 12 pt. font
- 1863
- 1865
- 2.1
- 2007)
- 2014
- 2015
- 2015). Through sizeable as well as creating cultivating
- 2021). HTML pages are an excellent example that allows network shares hence makes the embedding of the resources possible. Therefore
- 24(9).
- 25 Feb. 2017. Web.20 Mar. 2017.Students Turn to Canada in Trump ImmigrationEra. The Times-Picayune. TheWashington Post
- 4.1 The following gives the number of pints of type A blood used at Woodlawn Hos
- 4)."
- 40Spring 2015 OPRE3310: OPERATIONS MANAGEMENT HOMEWORK #6 Part I-Problems: 1
- 40Spring 2015 OPRE3310: OPERATIONS MANAGEMENT HOMEWORK #6 Part I-Problems: 1. Ma
- 5-1. McBurgerâs fast-food restaurant has a drive-through window with a single
- 5.12 The buyer for Needless Markup, a famous high end department store, must dec
- 500 (maximum) words (about 7-10 pages
- 500. How much total rental income must Sarah report this year?
- 7
- a ""loss of the will to fight."" The Confederacy did not lack ""the means to continue the struggle
- a medical di ct ionary
- A company uses 36,000 tyres to produce car wheels every year. The company can m
- A Confederate Lady Comes of Age: The Journal of Pauline DeCaradeuc Heyward
- A corporations __________ ultimately control decisions about its direction, v
- A family friend has a small winery in California. He has asked you to spend an a
- a matter of northern luck in finding someone who could do the job and southern misfortune in picking the wrong man? Before we come to this beguilingly simple conclusion
- a resource toolkit about A- EBPs that lists online resources available to LHDs [38]
- A shared resource, such as land, air, or water, that a group of people uses c
- A Systematic Study of Asset Management using Hybrid Cyber Security Maturity Model
- a U.S. computer parts manufacturer
- Ab104
- academic ability
- accessed11/April/2017[22] NMA
- Accounting
- acquire (at least) one initial Discourse. This initial Discourse
- Actions for Week 1 Discussion Topic 1Have you ever worked with anyone like Jenny
- acts
- adding beliefs
- Afroamer Hist
- After reviewing the assigned readings, write a paper of 1,000-1,500 words that c
- against first one Army and then another
- aggression
- Ahs6640
- Algebra
- All Questions Question 1. Question : IBM CEO Samuel Palmisano says that his com
- along with a description of any necessary actions the client should consider in view of your observations and findings.
- along with pre-linguistic
- along with the review and application of the concepts
- America
- American Military University
- an individual must combine four components of speech into an adaptable system of communication. These include elements of sound (phonology)
- An organization following effective HRD practices will have completed Phase I o
- analyze and evaluate the following questions:
- analyzing
- and academic grammar and usage.
- and adaptable attention.
- and all up the hillside
- and APA citations Value: 15 Pts
- and apply information
- and assist the Government in maintaining our freedom.
- and be sure to number them so you can effectively refer to them in your narrative. When doing so
- and cite and reference your sources to avoid plagiarism."
- and communication methods.Discuss in detail how this issue affects healthcare delivery and advanced nursing."
- and comparisons
- and ethical issues related to an issue such as a data breach
- and Ethics""à à Please respond to the following:Read the article titled â¬ÅCriminal Justice Ethics for Everyoneâ¬
- and following directions ('What did the bird do next?""). Phonological awareness activities included syllable segmentation
- and Gettysburg would have concluded that the South really wanted to lose. …
- and grammar are fundamental components of language acquisition
- and Historical Causes
- and identifies all organizational assets at risk to a variety of hazards.
- and inclusion.
- and industrial capacity were clearly overwhelming. According to the census of 1860
- and inspiring; however many teachers continue to underemphasize higher-level thinking for under stimulating
- and institutions.
- and logical security controls
- and often write
- and other issues that affect the curriculum.
- and other legal issues unique to the site
- and other references. (All references must be from professional and/or academic sources. Websites such as Wikipedia
- and Personal Conduct: Implications for Theory and Policyâ¬
- and personal health record (PHR)
- and politically less ""developed"" or modernized than the North in 1861 may not by itself fully explain why war had to come
- and possible assets
- and quarrelsome man with a fatal passion for always being in the right and for standing by his friends
- and related sources from the Internet."
- and related terms for two separate concepts. (examples: technology-computer
- and school can further the understanding of the impact on child development. The first case study seeks to help us understand the link between excessive television viewing and ADHD
- and scope of the paper. Value: 5 Pts
- and select one (1) country of your choosing from the drop-down menu. Review the six (6) cultural dimensions of the selected country. Next
- and self-management skills to succeed with complex tasks. Those who study this approach compare the human mind to a computer
- and sold them to Cuba; but we don't believe that.) We want to be placed on land until we are able to buy it
- and suffix. Then locate a new term f rom the chapter that uses the word part. Combining Forms Meaning Chapter Term Meaning
- and Sunday law enforcement declined as the century progressed. By the last quarter of the century
- and the consultant's general and specific research conducted about the organization selected for the project. The paper will total between 1
- and the library in particular as the security target.
- and the long
- and the long-term memory store. As information moves from one part to the next
- and the wounded are streaming to the rear by hundreds
- and their disposition to aid either side; state fully your views.
- and then closing your eyes. Your sensory register has just been activated. It took in a wide variety of new information; however
- and then of a very inferior quality. Soda
- and they are larger
- and this provides opportunities for criminals and fraudsters to conduct illegal activities without being detected. However
- and to pay the expense pecuniary cost of all this
- and transportation facilities
- and Transportation and Telecommunication. Using The World Factbook
- and what are your means of knowing the sentiments of those living in the country.
- and when I spoke to him a few days ago about carrying out his contract he told me that he was sorry he made such a bargain with us:
- and with glori ous old cheers
- and/or federal rules and regulations.
- Animation
- Answer in 5-6 sentences per question.Discuss the strategic importance of layout
- Answer the following questions based on this data: Work on a project has gone
- Anthropology
- any compari son involving the will to win of the respective sides cannot ignore the dif ferent situations that they faced. Except for Lee's brief forays into Maryland and Pennsylvania
- Applied Science
- Applied Sciences
- Archaeology
- Architecture
- Architecture and Design
- are different in each country. Basically
- are not very convincing either. The problem is that the North experienced similar internal divisions
- Arnold
- Art
- Art & Design
- Article Writing
- Arts
- as I understand it
- as opposed to a subjective
- as to the war
- As we move into the last two weeks of our stimulating, interactive dialogue, we
- as we will learn
- Ashford University
- Assessment Brief Assessment ObjectiveAfter getting through the materials of this
- Assessment item 2Individual case study All students are to submit electronicall
- Assessment question and detailMust be 1000 wordsMust include the below reference
- Assignment 2: Case StudyTraining AssessmentTraining assessment is an important t
- Assignment AssistancePlease see attached. 1 Presentation to Executive Team Imag
- Assignment Instructions Instructions: Mid Term Paper: Choose three of the
- Assignment Instructions Week 8 Assignment: This assignment is due at the end of
- Assignment Instructions Week Five Paper 3-5 page Paper For this assignment, you
- Assignment InstructionsInstructions: This week you are expected to write a resea
- Assignment InstructionsInstructions:This week you are to write a research paper
- assignment need help help onassignment need help help on
- Assignment OutlineSee Attached Assignment (3) (Outline Only) Communication Plan
- Assignment QuestionsExcel data entry and modeling is not introduced at this late
- Assignment To complete the following assignment, go to this week's Assignment
- Assignment To complete the following assignment, go to this week's Assignment l
- Assignment To complete the following assignment, go to this week's Assignment li
- Assignment: OperationsMake sure you have completed the Learning Activity in this
- Assignment: RecruitmentIn this week and next, you will be learning about human r
- Assignment: The Employment ProcessIn week two, you figured out what positions ne
- Assignment: The Supply ChainIn this Assignment you will practice with applying s
- Assignments
- assignments for wakassignments for wak
- Assistance with Assignment – Please see attachments
- Assistance with AssignmentPlease see Attached
- at first
- at least
- attention problems were more strongly related to inhibition than to IQ. According to Friedman and colleagues (2007)
- attitudes
- attributes change within and between stages to increase the efficiency with which children use their limited working-memory capacity. As children improve their abilities to process data
- Auburn University
- Australian
- Aviation
- BA207
- Bachelor Of Applied Social Science
- Banking
- Beauty Therapy
- because they had taken an active part for the rebels
- Before you begin this assignment, be sure you have read the UMUC Haircuts Case S
- Before you begin this assignment, be sure you: 1. Read the UMUC Haircuts Case S
- begins with the development is self-awareness
- behavior
- below is a list of possible topics for you to consider. If you have any questions
- Bethel University
- beyond the family and immediate kin and peer group. These may be 1111.
- Bhr 3352
- Bib355
- Binghamton University
- Biology
- Biology – Anatomy
- Biology – Ecology
- Biology – Physiology
- Blog
- Bls221
- Bob Carltons golf camp estimates the following workforce requirements for its se
- body
- Bos4025
- Boston University
- Bowie State University
- brings with it the (poten- u.il) acquisition of social ""goods"" (money
- Brookhaven College
- BSOP 326 Week 4 Midterm Exam 1. (TCO 6) An effective leadership system is desig
- BSOP 326 Week 8 Final Exam 1. TCO 1) Which of the following factors allowed Fre
- BSOP 334 Week 8 Final Exam Page 1 1. (TCO 1) Which of the following functions
- BSOP 588 Fianl Exam Page 1 1. TCO E) Determining how customers will value your p
- BSOP 588 Final Exam 1. (TCO E) Suggested reasons why many customer satisfaction
- BSOP 588 Final Exam 1.(TCO E) Which of the following is NOT TRUE about the Ameri
- BSOP 588 Managing Quality Week 1 Discussion 1, Definition of Quality Discussion
- BSOP 588 Midterm Exam 1. (TCO A) Discuss how a fast-food restaurant could measur
- BSOP 588 Midterm Exam Page 1 1. (TCO A) Compare and contrast the evolution of qu
- BSOP 588 Midterm Exam Page 1 1.(TCO A) Contrast the mindset of management under
- building description
- Bumg2017
- Bus 119
- BUS 520 Assignment 3 Part I: Leadership Style: How Are Leaders Leading in the 21
- Bus623
- Bushwood Supermarkets is a major food retailer in the Philadelphia area. The cha
- Busi 511
- Business
- Business & Finance
- Business & Finance – Financial markets
- Business & Finance – Marketing
- Business and Finance
- Business Applications, Analytical Decision Making, and Problem SolvingThe presen
- Business Chosen for assignments is: A Caribbean RestaurantBUS475 Week-1 Knowledg
- Business Finance – Accounting
- Business Finance – Economics
- Business Finance – Management
- Business Finance – Operations Management
- Business Plan: The Problem & AnswerAs a Team, pick a topic.You will describe you
- Business Strategy
- but also facilitates nosebleeds for some.The pharynx is a muscular
- but at least 80% of the required information has been included in the paper(4.125)Excel file has not been embedded correctly
- but cannot always recall or retrieve the word in order to communicate. In other words
- but had been unsuccessful. Galadriel encouraged him
- but has affected every other aspect of our lives.
- but it offers more information on what could possibly be done with specimens/data collected. These issues will continue to arise as future research consent is becoming more and more common.
- but not be limited to
- by Pierre LemieuxAfter the economists' analytical assault
- by the labor of the women
- Ca499
- Calculus
- California State University Fullerton
- can be studied
- can never 21 really be liberating literacies. For a literacy to be liberating it must contain both the Discourse it is going to critique and a set of meta-elements (language
- Case 1: GEs Two Decade Transformation: Jack Welchs Leadership-HBS # 9-399- 150Ca
- Case AssignmentTMSFor this Case Assignment, read the article below concerning tr
- Case Incident 3.2Tardy TomOn September 30, 2007, a large national automobile-lea
- Case StudiesUnit I Case StudyCase studies are an important learning strategy in
- Case Study #1: Planning and Organizing Students will read the case study that f
- CATEGORY
- Ch. 1 and Ch. 2 of Operations Management: â¢Problem 1-1 â¢Problem 1-2 â¢Probl
- Chapman University
- Chapter 3
- CHAPTER 3: PROJECT MANAGEMENT TRUE/FALSE 1. The ES of an activity that has only
- Chapter 4 Advanced Topics in Risk Management 1. All of the following are financi
- Chapter 7 Homework Problem 14.14. Mama's Stuffin' is a popular food item during
- Chapter 7 HomeworkProblem 14.14.Mama's Stuffin' is a popular food item during th
- cheaper manpower
- Chemistry
- Chemistry – Chemical Engineering
- Chemistry – Organic chemistry
- Chemistry – Pharmacology
- Chemistry – Physical chemistry
- Childcare
- Cis650
- CISY2320
- citations and references are present and APA standards are followed.
- Cj130
- Cjs 221
- Classics
- Classification of Food and Restaurant Images using CNNs
- Clayton State University
- CMGT 580
- Cod
- COLL 148 Critical Thinking COLL 148 Week 1 Journal COLL 148 Week 1 DQ 1 COLL 148
- COLL 148 Week 4 Quiz 1. Which of the following is NOT true about words: 2. Whe
- COLL 148 Week 6 Quiz 1. All of the following are true regarding planning for yo
- Collaborative Innovation Strategy
- College Of The Canyons
- Colorado Christian University
- Colorado State University
- Columbia College
- Columbia Southern University
- Com 230
- COM-FP3200
- Comm104
- Commerce
- Communication
- Communications
- Complete Learning Exercise D on p. 349 in your textbook. When complete, select t
- Complete Objective Questions 1, 2, 3, and 4 at the end of chapter 5 in the textb
- Complete Objective Questions 16, 17, 19, 20, and 21 at the end of Chapter 2 in t
- Complete Objective Questions 6, 7, and 8 at the end of chapter 4 in the textbook
- Complete the Analytics Exercise: Comparing Companies Using Wall Street Efficien
- Computer Science
- Computer Science – Java
- Computer Science- Python
- Concordia University
- Conduct an interview with someone who has a career or job position that is diffe
- Consider the following table of activities, completion times and predecessors
- Construction
- consultants will submit the "Risk Assessment/Security and Safety Planning Instrument" (work product)
- Consultative (C1)
- Corporate strategy is concerned with the broad and long-term questions of what b
- correctness
- Course 1151 – MAN4600 – International Management – Section RVE – Spring 20
- Course Operations Management Test Quiz 1 Instructions This quiz consist of 30 mu
- Course Project: Beginning or End of Unions? Submit your project to the Dropbox l
- Course Project: Course Project: Benefit Plan Design Analysis Objectives The
- Course Project: Course Project: Benefit Plan Design Analysis Objectives The C
- Course Project: Leadership and Organizational Behavior in ActionObjectiveResearc
- Course Textbook Spiro, R. L., Rich, G. A., & Stanton, W. J. (2008). Management
- Coursework
- Criminology
- Crosbie
- Cs330
- Cultural Studies
- culturally appropriate intervention to address childhood obesity in a low-income African American community.
- Current LocationTake Test: Exam 3Assistive Technology Tips [opens in new window]
- Cuyamaca College
- D. Craig Exploration Company has been offered a lease to drill for oil on a part
- Data Analysis
- DC Delco CorporationDC Delco Corporation manufactures and sells the various elec
- decide the extent to which each stage of the performance management process is relevant to employee̢¢s performance. Justify your response.
- Decision Making Problem Solving
- declines in reading ability and creative thinking
- Depaul University
- describe how children acquire
- Design
- Details: Complete Objective Questions 1, 4, 10, 11, 13, and 18 at the end of cha
- Details:Complete Objective Questions 12 and 17 at the end of chapter 16 in the t
- DEtails:Complete Objective Questions 17, 21, and 40 at the end of chapter 20 in
- Details:Complete Objective Questions 7 and 10, at the end of Chapter 12 in the t
- Details:Complete Objective Questions 7 and 8 at the end of chapter 8 in the text
- Details:Complete problems 1.1, 1.9, and 1.13 in the textbook.Submit one Excel fi
- Details:Complete problems 11.5, S11.4, S11.9, S11.15, 12.1, 12.4, 12.13, and 12.
- Details:Complete problems 3.11 and 3.17 (parts c, d, e, and f) in the textbook.S
- Details:Complete problems 4.1, 4.3, 4.5, 4.25, and 4.27 in the textbook.Submit o
- Details:Complete problems 6.12, S6.11, S6.20, S6.23, S6.27, and S6.35 in the tex
- Details:Complete problems 7.5, 7.7, and 7.11 in the textbook.Complete supplement
- Details:Complete problems B.1, B.5, B.7, B.11, and B.21 in the textbook.Submit o
- Details:For this assignment, you will discuss how Christian principles can be ap
- Develop a Training or Intervention Strategy to Address the Needs Creating a deve
- Development of insulin resistant obese rat modelto evaluate the efficacy of Single Chain Insulin (SCI) analogs
- Devry HRM599 final exam Page 1 Question 1. 1. (TCO A) Many employees feel as tho
- DEVRY MAMT404 ALL DISCUSSION PAYMENT
- devry mgmt 404 quiz downpayment
- Devry MGMt404 week 4 quizDevry MGMt404 week 4 quiz
- devry mgmt404 week 4 quizQuestion 1.1.(TCO 1) Which document is created during t
- devry mgmt404 week 7 quiz
- Discuss how employers might reduce costs in some of the major benefits areas in
- Discuss how you can use VCA, RBV, and SWOT analysis to gain a stronger sense of
- Discuss several advantages of using the M/M/1 waiting line queuing model. Clearl
- Discuss what your experiences have been with new orientation or onboarding proce
- discussion week 1Read Case Study 3.1 The Keflavik Paper Company is a case with a
- Discussions To participate in the following discussions, go to this week's Dis
- Discussions To participate in the following discussions, go to this week's Disc
- Discussions To participate in the following discussions, go to this week's Discu
- DISCUSSIONSWEEK 1 Striking a Balance (graded) When efficiency, equity,
- DISCUSSIONSWEEK 1discussion week 1Read Case Study 3.1 The Keflavik Paper Company
- DISCUSSIONSWEEK WEEK 1 Effective IMC World (graded) IMC enables companies
- discussionWhat does it mean to say that managers should maximize shareholder we
- distribution
- Distribution Center is a facility or a group of facilities that perform consolid
- Dmm613
- Do the process and actions associated with "Mental Mastery" preclude someone fr
- Do you think that cognitive abilities should be part of the police exam? Do you
- Drama
- Due Date: May 25, 2014 23:59:59 Max Points:45Details:Complete problems 9.11, 9.1
- Due Wednesday, 100 PointsComplete the self-assessment (this is about your work v
- Due Week 5: Risk Management PlanRemember, risk is an uncertain event or conditio
- each time a user extracts the ZIP file
- Earth Science – Geography
- Earth Science – Geology
- eating
- Ece 312
- Ecom301
- Ecommerce
- Economics
- Economics For Managers
- Education
- EDUS has decided to acquire the institution and needs to establish a management
- Effect of Anti-obesity (orlistat) on Type 2 diabetes Mellitus remission in patients in Saudi Arabia.
- Effective Organizational Design" Please respond to the following: From the case
- elasticity
- Elsa Treebeard
- Embry Riddle
- Emglish
- EMGT 631: Production and Inventory ModelingMini Project 3Consider the jobs in th
- employees
- Employment
- En206
- Eng 100
- Eng-106
- Eng225
- ENGH302
- Engineering
- Engineering – Civil Engineering
- Engineering – Electrical Engineering
- Engineering – Electronic Engineering
- Engineering – Mechanical Engineering
- Engineering – Telecommunications Engineering
- Engl311
- English
- English – Article writing
- English 361
- English Language
- English Literature
- Environment
- Environmental Science
- Environmental Sciences
- Environmental Studies
- Envisioning a BusinessIn this Assignment you will be asked to use your imaginati
- ESAs Rosetta Cometary Rendezvous Mission
- especially for a small company
- Essay Help!(see attached)Identify and describe a recent crisis or controversy th
- Essay Writing
- essential questions (at least 3 pe. r unit)
- Estate Management
- etc¦). Please note at least five organizational activities and be specific when responding.
- European Studies
- evaluate the test or the tool's validity and reliability
- even if they do not yet comprehend the symbolic function of the elements of print.
- every battle
- Excelsior College
- Exercise for Uncertain Demand In a given period demand for an item is equally
- Exercises: 13.1, 13.3, and 13.11The followingexercises are required and cover to
- expansion of distribution channels
- Explain what ismeant by the term Strategic Planning Gap and critically evaluate
- explore this nuanced question: Did the United States win the war
- Exports are: all goods and services sold abroad and sent out of a country
- Expression and Purification of a novel recombinant form of UDP-N-acetylenolpyruvoylglucosamine reductase (MurB) for structural and functional studies
- Family
- farmers
- Fashion
- FILL In THE BLANK117. A(n) ____________ is an operation that has the lowest effe
- FILL-IN-THE BLANK71. ____________ is an organization formed to ensure that progr
- Film Industry
- Film Studies
- Fin 500
- FINAL EXAM MGT 5002 MULTIPLE CHOICE CHAPTER 9 (9-5) Required return 1). If
- Final Paper To complete the following final paper, go to this week's Final Pape
- Final Project You will complete a Final Project in which you will analyze an org
- Final Report: Growth, Innovation, Sustainability, and Succession (30%)The instru
- FINAL TEST MM5009 DECISION MAKING AND NEGOTIATIONINSTRUCTIONSA. Personal refle
- fire protection systems
- Florida Gateway College
- Florida International University
- Focus of the Final Paper Prepare an 8- to 10-page fundamental financial analys
- FolderWritten Assignment 1InstructionsInstructions: Locate an article describing
- For each of the roles of HR (Administrator, Enforcer, Expert and Strategic Busin
- for Sickles has been borne from the field minus one of his legs
- For the first four Units of this course, you have learned several topics in disc
- Foreign Languages
- Foreign Languages – Spanish
- forestalled European mediation and recognition of the Confederacy
- forumsweek 1Take some time to discuss, in your opinion, the most interesting ma
- From an organization/firm perspective, why are talent management, leadership, a
- from https://www.pcmag.com/news/fat32-vs-ntfs-choose-your-own-format
- frontier conditions
- Geb3213
- General Studies
- Geography
- Geol100
- Geometry
- George Brown College
- Gooderham
- Government
- Grade Details – All QuestionsPage: 1 2Question 1. Question : Which of the follow
- Grade Details – All QuestionsQuestion 1. Question : During 2015, NICO Corporatio
- GradingProceduresThis paper should be a research paper that is a minimum of 10pa
- Grand Canyon University
- Grantham
- Grantham University
- Grossmont-Cuyamaca Community College District
- GSCM 530 Week 1 Homework assignmentAssignmentAssignment: page 431, Problems
- GSM-730: GLOBAL STRATEGIC MANAGEMENTFinal PaperOVERVIEW:Strategic management is
- GU299
- Hate Crime Action Plan Fails To Address Islamophobia TheMuslim News (The Muslim News
- He 553
- he commonly exhibited an indomitable sense of personal independence. The non-slaveholder
- he expanded the regular army
- Head Start)
- Health & Medical
- Healthcare
- Hi225
- Hillsborough Community College
- Him301
- His 202
- his wife’s biggest fear came true—their daughter was removed from the home and placed with his parents.
- Hist1122
- Hist2020
- History
- History – American history
- History – Ancient history
- History – World history
- HLS3302
- HLTH 642
- Home Depot
- Homework #4 Problem 1 The management of a factory is going to establish a main
- Homework Download the Terms to Know document that is in Doc Sharing. Pick
- Homework Please find an article related to government-mandated benefits (un
- Homework Please find an article related to healthcare programs. To help loca
- Homework Please find an article related to pension programs. To help locate
- Homework Review the Case application: APPRAISING THE SECRETARIES AT SWEETWAT
- HomeworkComplete the following problems from Chapter 4 in your text. The Homewor
- HOSP 585 Week 4 Midterm Exam 1. (TCO A) Which of the following is not a common
- HOSP 585 Week 8 Final Exam 1.(TCO F) You are the vice president of purchasing f
- Hospitality
- hostile
- Housing
- how Britain attempted to resolve those problems
- how many trout should Ernie purchase from Denver each week? *Show work with Excel* "
- how much interest expenditures would Durham City recognize related to these bonds?
- however
- HR Management
- HRM 586 Final Exam 1. (TCO A) You are the new leader of the local union at your
- HRM 586 Final Exam HRM 586 Page 1 1. (TCO A) You are the new leader of the loca
- HRM 586 Labor Relations Week 1 DQ 1, Fighting Employer's Premises DQ 2, Union
- HRM 586 Week 8 Final Exam 1. (TCO A) How do public-sector employees rights and i
- HRM 587 Final Exam 11.(TCO All) For the next set of questions, you will first se
- HRM 587 Managing Organizational Change HRM 587 Week 1 Course Project Managing Or
- HRM 595 Final Exam 1. Joe McDonald is the HR manager of ACME chemicals. His bos
- HRM 595 Negotiation Skills WEEK 1 Assignment; Personal Bargaining Inventory Ans
- HRM 598 Compensation Week 1 Discussion Question 1, Compensation Definitions and
- HRM's Strategic RoleHuman Resource positions are called "staffing" (not "line")
- HRM355BAProgrammed instruction (PI) is a method of self-paced learning managed b
- HRMT 603
- Hsl2301
- Hst 300
- HUM 176 WEEK 4 Social media networks essay Write700- to 1,050-word paper and ad
- HUM 301
- Hum420
- Human Resource Development HRA-539 discussion and assignments needed Module 1: T
- Human Resource Management
- Human Resource Management Human resource management is ? a.comprises the con
- Human Resources
- Human Rights
- Humanities
- HW for Module6Financial Management Homework (Chapter 7: Stocks and Stock Valuati
- I have a 40 question exam I need help with I can only post 1/2 the questions1. Q
- i need the attached work to look like this. please redo and make it look like this.
- I. SW Co. MACRSII. Jones_DepreIII. Impact_Bus_orgIV. SaratogaV. ROCI_EVAVI.Ope
- Ibsu487
- Identify a current organization that is not, in your opinion, a Learning Organi
- identify the leadership theory that best aligns with your personal leadership style
- identifying the hand of Providence in American history
- if adopted
- if indeed he come back alive. May God's providence interpose to prevent his going! His presence is surely needed at home; his hands are taken away by the militia draught
- if you suggest trying to do this
- Iii Mlch
- III nuistery of such superficialities was meant to
- Im looking for a 4 page research paper APA format, double-spaced pages, on the
- Implementation of Education in Sustainable Materials into Civil Engineering Undergraduate CurriculumDecember 12th, 2018
- Improving Efficiency Write a 2-page paper describing how trade, comparative adv
- In a final paper, respond to the questions below to demonstrate your overall kno
- In a production run of 300 units, there are exactly 20 defective items and 280
- in almost all other areas
- in any other way
- in districts
- in my opinion.
- in the Associationof Southeast Asian Nations Website
- In this module you've been reading about the staffing functionthe process of rec
- In this Session Long Project, you are asked to select one of the following organ
- In Unit 3, you will complete the next section of your final project, Organizatio
- In what generational cohort do you belongMillennial, Gen X, Baby Boomer, or Sile
- Inc.
- including information on the introduction
- indeed
- India
- Indiana University Bloomington
- Industrial Technical College
- Information Systems
- Information Technology
- INFS519
- initially able to muster only 190
- International Business
- International Relations
- International Studies
- Internet
- Introduction To Analytics
- Introduction To Philosopy
- INVENTORY MANAGEMENT 1. Number 2 pencils at the campus bookstore are sold at a
- is called
- is said to promote appreciation of language as a communication tool. This is distinguished from the phonics approach
- is the feeling of the colored people about enlisting and serving as soldiers of the United States
- issue(s)
- It Research
- it was a question whether our numerical strength was not more than balanced by these [dis]advantages.
- It490
- John Ruskin
- JOURNAL WEEK 2Journal To complete the following journal entry, go to this week'
- Journalism
- Juffer
- Kaplan University AB 203, Fall 2014 Managing Talent: Can yahoo still attract tec
- Kroger
- Labor Relations Paper Your first written assignment is due this week. The en
- Lakshman
- Languages
- later
- Law
- Law – Civil
- Law – Criminal
- Law2001
- Law710
- Ldr600
- Leadership
- leading to a decrease in aggregate demand. Which pair of factors contributed to this decline in wealth?
- leading to a decrease in consumer spending. Which of the following factors caused this decrease in consumer sentiment?
- learning and assessment.This chapter will discuss theories
- leaving their homes and undergoing suffering. I did not think there would be so many; the number surpassed my expectation.
- Lee
- legal and political institutions.' This new interpretation soon dominated popular attitudes.28(Links to an external site.)Links to an external site.
- Legal Regulations Compliance And Investigation
- Leisure Management
- Letourneau University
- Liberty University
- Lightspeed: An Adaptive Bilateral Negotiation Strategy
- lIlgll.Igt· (1II1In·d
- Lincoln's remarkable abilities gave him a wide edge over Davis as a war leader
- Linguistics
- List and briefly describe the two (2) reasons given in your textbook for why dev
- Lit 2000
- literacy and math experiences did you have while growing up? Based on what you've learned
- literacy is always plural: literacies (there are many of them
- Literature
- localism
- Located alongside a cobblestoned street in Old City, Old City Photographics (OC
- Logistic Management For Disasters
- LSS5100 Entire Course Unit I, Unit II, Unit III and Unit IV(***** All 4 Unit + O
- Lululemon in FinlandTABLEOFCONTENTS PAGE INTRODUCTION 3PROJECT OVERVIEW 4GLOBAL MARKET ASSESSMENT 8MODE OF ENTRY 12CULTURE 13ETHICS 15RISK AND REWARDS 16CONCLUSION 17REFERENCES 18APPENDIX 20
- MA; Cengage Learning.
- Ma105
- Ma225
- Mahometanism
- Major Problems in the History of the American South (Lexington
- makes answers to inquiries as follows:-
- Mana 3300060
- Management
- Management Planning in businessManagement Organizing in businessLeadership and M
- Management Theory and Practice In a four- to five-page paper (excluding title pa
- Marketing
- Maryland. The building has high visibility since it is situated near major thoroughfares
- Math
- Math144
- Mathematics
- Mathematics – Algebra
- Mathematics – Calculus
- Mathematics – Geometry
- Mathematics – Numerical analysis
- Mathematics – Precalculus
- Mathematics – Probability
- Mathematics – Statistics
- Mathematics – Trigonometry
- Mathematics SLDerivation and Geometry of the Catenary CurveTable of ContentsIntroduction1The Catenary Curve1-2Geometry and Defining Variables3-5Derivation5-7Application8-9Conclusion9Bibliography9-10
- Matu203
- MBA 540 Mid-term Exam 1. (10 pts.)Stella Ann Freeman is having a difficult tim
- MBA 595 – Leadership Project Guidelines The Leadership Project requires each s
- MBA 595 leadership in organization paper MBA 595 – Leadership Project Guidelines
- MBA 599 Strategic Management Case Project This capstone course requires each
- MBA 6133 PROJECT MANAGEMENT (ASSIGNMENT 1) Question No. 1 How would you def
- Mba5401
- MBALN609Assignment 2DUE DATE: END OF WEEK 7 (50% of Final Grade)Part 1Research t
- meaning
- Mechanics
- Media
- Medical
- Medical Essays
- meeting family and friends
- MGMT 303 Final Exam 1. (TCO1) Discuss the functions of management. Which functio
- MGMT 303 Midterm Exam 1.(TCO 1) The _____ approach of going green is a good exa
- MGMT 303 Principles of Management MGMT 303 Week 1 Week 1 Checkpoint (12 Question
- MGMT 303 Week 1 DQ 1 Who Is a ManagerMGMT 303 Week 1 DQ 2 Managerial EthicsMGMT
- MGMT 303 Week 8 Final Exam 1 1. (TCO1) A CEO of a local newspaper has noticed th
- MGMT 340 Business Systems Analysis Week 1 Course Project, Chapter 2 Problems an
- MGMT 340 Final Exam Page 1 1. (TCO 1) When developing information systems, an or
- MGMT 410 Human Resource Management Week 1 Strategic Linkages Assignment DQ 1 Th
- MGMT 410 Midterm Exam 1. (TCO 1) One of the implications of technology in HRM i
- MGMT 410 Midterm Exam 1. (TCO 1)HRM typically provides which of these types of t
- MGMT 520 Final Exam 1. List any bases Robins & Robins could sue Casings, Inc., u
- MGMT 520 Final Exam 1. TCO D Short Answer Question and Facts for Page 1 Question
- MGMT 520 Final Exam Page 1 TCO D. Questions: A well known pharmaceutical company
- MGMT 520 FINAL EXAM Week 8 1. (TCOs G and I)In the 1930s, after immigrating to
- MGMT 520 Legal, Political and Ethical Dimensions of Business WEEK 1 Discussion Q
- MGMT 520 Midterm Exam 2TCO B. Infuriated when Harry Reid is re-elected during th
- MGMT 520 Midterm Exam 31.(TCO B). Infuriated when Harry Reid is re-elected durin
- MGMT 520 Week 8 Final Exam 1. TCO D A well known pharmaceutical company, Robins
- MGMT 520Midterm Exam 1. (TCO I) Marianne Jennings wrote an article, Why an Inter
- MGMT 553 EXAM 3Part I: True /False (15 Points) 1. An extraordinary item appears
- MGMT 601 week1 DQ1 Executive Manager Q: Imagine yourself as the executive manage
- Mgmt 615
- MGMT E-2000 Fall, 2014 Problem Set 4 Answers (100 points total) All calculation
- MGMT E-2600 Spring 2015 NAME: ____________________________ last first . AVT
- MGMT404 quiz 7 Question 1.1.(TCO 4) An example of bottom up estimating wo
- MGMT600-1404A-04 Applied Managerial Decision-MakingTask Name: Phase 1 Individual
- MGMT640 Section 1121 Final Exam Summer 2014 Name: __________________________ D
- MGT 190 Exam 2 Question 1 The probability of collecting an outstanding account r
- MGT 321 System Build Project For this project, you will design a system for the
- MGT 4479-Management Seminar Mid-Term Exam-Parts 1-8Name_______________________1.
- MGT 505 WK 3 Assignment 1 – Communication Process Find a Web site that has an Ab
- MGT-655 | Inventory Management Module 8 DQ 1 Pick a particular company. Based on
- MGT-655 | Operations and Supply Chain Management Module 1 DQ 1 With respect to a
- MGT-655 | Operations and Supply Chain ManagementModule 1 DQ 1With respect to a f
- MGT-655 | Quality Management Module 7 DQ 1 Compare and contrast the characterist
- MGT160-SP. INTERNATIONAL BUSINESS (MGT160-SP)> TAKE ASSESSMENT: FINAL EXAM Take
- MGT190-SP. ENTREPRENEURSHIP (MGT190-SP)> TAKE ASSESSMENT: MGT 190 EXAM 2 NameMGT
- Mgt201
- Mgt211
- MGT404 quiz 7 If it cost two dollars today to buy a server, then it will
- Mgt630
- Mgt660
- Microgrid Energy Management Using a Two Stage Rolling Horizon Technique for Controlling an Energy Storage System.
- Middle East College
- Migration of Health Workers from Ghana Are financial incentives enough to stem the tide?
- Military
- Military Science
- MMHA6500
- Mmpa 6910
- MOD 5 Discussion How is cost-volume-profit analysis useful? MOD 6Dis
- mod 5 Discussion Question Do you believe the present rights given to strikers b
- mod 5 dq 1Review the Noodles and Company Service Process Design video at http
- mod 7 Discussion Question What are the steps both parties' advocates sh
- MOD 7 Module 7 Discussion Please give an example of a company using a
- Mod445596Id
- Module 1 – Case DISCRIMINATION, RETALIATION & THE LAW Developing and Enforcing A
- Module 1 – SLP STRATEGIC IMPORTANCE OF HUMAN RESOURCE MANAGEMENT For the Sessi
- Module 1 Discussion How do the major activities and systems of HR workers
- MODULE 1 DQ 1 Max Points: 5.0 With respect to a firms supply chain design, what
- MODULE 1 DQ 1Max Points: 5.0With respect to a firms supply chain design, what ar
- MODULE 1Module 1 Discussion Question 1 Would you be willing to relocate overseas
- Module 2 – Case MANAGING GROWTH AND DOWNSIZING Forbes Magazine offers a "layoff
- Module 2 – CaseHIRING PRACTICES & LEGAL COMPLIANCEImportance of Background Check
- Module 3 – Case MANAGING PRODUCTIVITY THROUGH JOB DESIGN AND WORK FLOW After rea
- Module 3 – CaseORGANIZINGAssignment OverviewZappos Shoes, led by young CEO Tony
- MODULE 3 Module 3 Discussion There are many benefits and costs asso
- Module 4 – Case INTERNATIONAL HRM Case Assignment For this Case Assignment, you
- Module 4 – CaseLEGAL CONSTRAINTS ON EMPLOYEE BENEFITSFMLA Leave FrustrationsIn t
- Module 4 – CaseStrategic Management: Internal Analysis and SWOTIn Module 4 Case
- Module 5 – Case EVALUATING HR SYSTEMS After reading the background materials, yo
- Module 5 – CaseLABOR LAW; EMPLOYEE SAFETYHyatt Regency Employee DiscontentTo beg
- Module 5 – CaseStrategic Management: Strategic Choices and ImplementationCase As
- Module 5 Discussion Question 1 An exchange rate is the price of currency. Google
- Module 5 DQ 2 Identify how production processes are organized in a typical manuf
- MODULE 5Module 5 Discussion In light of the oil disaster in the Gulf
- Module 6 DQ 1 Discuss the strategic importance of layout decisions. How can a pa
- MODULE 7 DQ 1Max Points: 5.0Compare and contrast the characteristics of Total Qu
- module3 dq 1Comment on the project management profession. What business areas c
- Montclair State University
- more reliable income to investors than the same firms common stock
- most adults can remember a list of about seven numerical digits. However
- most Civil War battles were fought according to the outdated tactical principles that generals on both sides had learned at West Point. In these very conventional battles
- Multiple Choice1. The _____ is the process used to identify user requirements, e
- MULTIPLE CHOICE19. The focus for a process improvement exercise should be on bal
- MULTIPLE CHOICE21. Which of the following statements regarding Bechtel is true?a
- multipronged offensive launched by Grant in 1864
- Music
- Music1407
- must name either the shape or the color
- n
- Name Midterm ExamInstructions Multiple Attempts This Test allows 2 attempts. Thi
- Name Midterm ExamInstructionsMultiple Attempts This Test allows 2 attempts. This
- Name the factors that may contribute to the global business failure of an organi
- nearshoring domestically can decrease global economies and restrict value for firms and international exchange of ideas (Peng
- NEED SLP for Module 2 through 5.See attached.Need 2 and 3 by 23 Feb, 4 by Mar 9a
- Need to complete a paragraph between 875-1090 words based on our team opening a
- needs to be between 250-300 words. What knowledge,traits, behaviors, and attitu
- no mat ter what their offence or to what section they belong
- no more
- no workable ""affirmative action"" for Discourses: you can't 19 Ill' let into the game after missing the apprenticeship and be expected to have a fnir shot at playing it. Social groups will not
- none are required in this Alternate Final Project. Since consultants cannot make personal
- not only capitalized on its initial advan tages during the war but was able to multiply them. In fact
- not writing)
- Nsg416
- Nsg451
- NSG6102
- Numerical Analysis
- Nurs 6051
- Nursing
- Nutrition
- OBJECTIVE1. Apply concepts and principles of project management to a specific pr
- ObjectiveResearch shows that people learn effectively when working on real probl
- observe
- occupy
- odule 1 – Case STRATEGIC IMPORTANCE OF HUMAN RESOURCE MANAGEMENT For the Case As
- of a relatively safe stock.c. The most appropriate risk-free rate
- often car ried out by women
- Olive oil made in Italy and sold in the United States is an example of which
- open communication
- Operation Security
- Operations Management Homework Problem 1: You are the newly appointed assist
- Operations Management II 73-431 Winter 2008 Odette School of Business Universit
- Operations ManagementHomeworkProblem 1:You are the newly appointed assistant adm
- OPS 571 Final Exam (****** 28/30 Correct *****)———————————
- or academic references from the Internet to answer the questions. Citations are not required for this homework assignment; however you may want to use them in order to answer the questions."
- or any
- or do those companies have an ethical obligation to protect people? In this assignment
- or perish there where they are doing so well
- or thinking out a sequence of acts ahead of time and allocating attention accordingly to reach a goal. While even infants demonstrate a basic aptitude for planning
- Organisations
- organization
- Organization Causal Loop Diagram For the fourth section of your Term Project you
- Organization Learning Disabilities For the second section of your Term Project
- Organizational Behavior Principles
- ORGANIZATIONAL THEORY, DESIGN AND THEORY (7TH ED.) 2013 JONES, G.R., PRENTICE HA
- Other
- out of employments in which the people of the community are associated
- Page 1 Question 1. 1. (TCO 1) Explain the employment-at-will doctrine. How doe
- Page 1 Question 1. 1. (TCO A) Many employees feel as though they are entitled
- pages of summary of the whole article (gnarl summary) I want4-5 pages of analyzi
- Park University
- Part 2A: Final Reflection ComponentIn our final reflection and planning paper yo
- Part I Quality Control (11 points) Southwest keeps gate time of its planes
- Partnership And Corporate Taxation
- perceptions
- perhaps
- Personal Development
- personal property loss/damage
- Persuasion And Social Movements
- PHASE 1Reminder: Initial Discussion Board posts due by Wednesday, responses due
- phase 1SCM210-1501A-03 Introduction to Logistics/Supply Chain Management Task Na
- Phil 112
- Philosophy
- Philosophy 127B
- Photography
- Physics
- Physics – Electromagnetism
- Physics – Geophysics
- Physics – Mechanics
- Physiology
- PJM 6025 – Project Scheduling & Cost PlanningInstructions Assignment No. 3: Dura
- Platform as a Service (PaaS)
- Please see attached document
- Please see attachementsPlease see attachements
- Pm598
- political developments are in another
- Political Science
- Politics
- PowerPoint slides
- practitioners interviewed were selected by the director and this could introduce selection bias. Finally
- Primary Discussion Response is due by Wednesday (11:59:59pm Central), Peer Respo
- Principles Of Business Management
- Prob (D>Qo) >75.00-25.00/100.00-25.00 > Prob (D> Qo +1)How I get D>Q and
- Problem 1 Dr. Jack is in charge of the Blood Bank at the local hospital. Blood i
- Problem 1: Specialty Contractors, Inc. In early 1993, Specialty Contractors, Inc
- procedural
- produce sounds
- Programming
- PROJ 592 Project Cost and Schedule Control Week 1 You Decide Project Cost Sche
- PROJ 592 Project Cost and Schedule Week 1 You Decide Project Cost Schedule Week
- Project 1 ProblemFor this project you can use EXCEL for calculations and then cu
- PROJECT DESCRIPTION Jennifer Christie, a licensed physical therapist, opened th
- Project Management
- Project Management Questions1. What are possible sources of conflict within a p
- Project Management Questions1. What are possible sources of conflict within a pr
- Project Paper Assignment (Linear Programming Models) Page 1 of 2 This is a ch
- Project Schedule and BudgetA project plan cannot be considered complete until th
- project title
- pronunciation expands
- Property
- provide a discussion on what could have been done better to minimize the risk of failure. If you have not yet been involved with a business process redesign
- Psy3380
- Psy570
- Psych620
- Psych635 Psychology Of Learning
- Psychology
- punctuation
- Purdue Global University
- Purdue University
- Q1. To decrease the nation's money supply, the Fed can increase reserve requirem
- Question 1 – Project Management (6 points) Here are the precedence requirements,
- Question 1 (1 point) .umuc.edu/d2l/img/0/QuestionCollection.Main.infQuestionUnsa
- Question 1 1 / 1 point What is the future value of $1,000, placed in a saving a
- Question 1 1 / 1 point Which of the following cannot be engaged in managing the
- Question 1 1 points Save The most typical time frame for a budget is
- Question 1 1 points SaveThe most typical time frame for a budget isone month.thr
- Question 1 Not yet answered Marked out of 20 Flag question Question text Organiz
- Question 1 of 10 10.0/ 10.0 Points According to the text, how many activities ar
- Question 1 of 10 10.0/ 10.0 Points An organization following effective HRD pract
- Question 1 of 10 10.0/ 10.0 Points Secondary HRM functions include? A.Compensa
- Question 1 of 1010.0/ 10.0 PointsAn organization following effective HRD practic
- Question 1 of 20 0.0/ 0.25 Points One advantage of a general purpose job board
- Question 1 of 40 0.25/ 0.25 Points ______________ were granted by
- Question 1 of 40Which of the following options is most likely to be used for rea
- Question 1 The advent of project management has been most profound in Answer Aut
- Question 1 The reorder point in a continuous review system is ________. Answer
- Question 1 To facilitate clinical support services ability to benchmark and
- Question 1. 1. Identify the steps in the organizational change process, and des
- Question 1. 1.In a PERT network, the earliest (activity) start time is the: (Poi
- Question 1. Question : Do you take care of your children or have other respons
- Question 1. Question : Using concepts from your text, outside sources and your o
- Question 1.1.(TCO 1) Different levels of planning in supply chain operations man
- Question 1.1.(TCO 1) Which document is created during the initiation
- Question 1.1.The maximal-flow technique might be used: (Points : 3) to help desi
- Question 1(1 point) .gif" alt="Question 1 unsaved"> Soaring Eagles Corp. has to
- Question 10 out of 10 points Do NOT round off your numbers to integers.After
- Question 11-1 Describe in general terms, how you think the distribution system,
- Question 2: (13 points) A manufacturer of greeting cards must determine the orde
- Question 2: (13 points)A manufacturer of greeting cards must determine the order
- Question Completion Status:12345678910111213141516171819202122232425262728293
- Question Offered Price $160.00 Trident LOG
- quizesQuestion 1 of 10 10.0/ 10.0 Points Examples of specific employee benefits
- R&D
- re ceived at these Head Quarters. To these latter I refer you for all minor details of operations and battles.
- Read the articles on aging below. Develop a 3-5 page paper on Aging in the Workf
- Reading
- reading performance will suffer.
- recognize it as a system
- recorded the struggles on the battle field of Gettysburg. Franklin Haskell
- Reference this
- regardless of season and weather
- Regent University
- region
- regulation of accounting/financial markets
- relatedness
- Religion
- Reminder: Initial Discussion Board posts due by Wednesday, responses due by Sun
- Reminder: Initial Discussion Board posts due by Wednesday, responses due by Sund
- requiring the organization's urgent action and those less critical and/or less likely to occur that may be addressed later. Note: To enhance the presentation of the risk assessment findings
- rescreening (insider threats)
- Research and write a 5-7 page paper and 10-12 slide power point on Interviewing.
- respi rat i ons 24 and l abored
- Retail
- Review Test Submission: Q02 Course Intro To Management Science (Winter 2015)Test
- Review Test Submission: Q04Course Intro To Management Science (Winter 2015)Test
- Review Test Submission: Q05 Course Intro To Management Science (Winter 2015)Test
- rhyming
- Risk Management (graded) Assume you have just been assigned to a project risk te
- Rsch8460
- RWS280
- safety statutes
- SAINT GBA440 MODULE 1 ASSIGNMENT – CASE STUDY 1GBA440Module1 Introduction to the
- SAINT GBA440 MODULE 3 ASSIGNMENT 2 – RESEARCH PAPERGBA440Module3 Politics, law ,
- Saint Leo MGT 325 Finance for Managers Unit 8 – Term Paper/Project 2015
- Saint Leo MGT 325 Finance for ManagersUnit 4 Test 1Grade Details – All Questions
- SAINT MGT430 MMGT 430Module 4 Business in a Public Policy Case Study 4 For this
- SAINT MGT430 MODULE 1 CASE STUDYSAINT MGT430 MODULE 1 CASE STUDYMGT 430Module1 I
- SAINT MGT430 MODULE 2 CASE STUDYSAINT MGT430 MODULE 2 CASE STUDYMGT 430Module 2
- SAINT MGT430 MODULE 3 CASE STUDYSAINT MGT430 MODULE 3 CASE STUDYMGT 430Module 3
- SAINT MGT430 MODULE 5 CASE STUDYMGT 430Module 5 Business and the Natural Environ
- SAINT MGT430 MODULE 6 CASE STUDYMGT 430Module 6 Business and Technology Case St
- SAINT MGT430 MODULE 7 CASE STUDYMGT 430Module 7 Business and Stakeholders Case
- SAINT MGT430 MODULE 7 TERM PAPER
- SAINT MGT430 MODULE 8 CASE STUDYMGT 430Module 8 Business and Stakeholders (conti
- saint mgt441 module 1 assignment 1MGT441Module 1 Introduction to Labor Relations
- saint mgt441 module 2 assignment 2MGT441Module 2 Union Structure , Membership ,
- saint mgt441 module 3assignment 3MGT441Module 3 Introduction to bargeraining
- saint mgt441 module 5 assignment 4MGT441Module 5 Contract Negotiations and Impas
- saint mgt441 module 6 assignment 5MGT441Module 6 Union Avoidance Strategies and
- saint mgt441 module 7 project paper MGT 441 Project Paper Guidelines Instructi
- Sales and Operations Planning (graded)Explain the sales and operations planning
- Saudi Electronic University
- Savannah State University
- saw what was happening
- Scheduling departmental manpower for a project is a very difficult task, even if
- Science
- Sciences
- SCM210-1501A-03 Introduction to Logistics/Supply Chain Management Task Name: P
- SCM210-1501A-03 Introduction to Logistics/Supply Chain Management Task Name: Ph
- SCM210-1501A-03 Introduction to Logistics/Supply Chain Management Task Name: Pha
- Sears
- Security
- self-actualization
- several things can happen
- SHORT ANSWERS129. Explain why a bottleneck limits system output.130. Describe a
- skills
- SLp 3 STRATEGIC MANAGEMENT: EXTERNAL ANALYSIS AND SWOT An external analysis is v
- so that it is not biased?
- so too
- Soc121
- Soc201
- Social Policy
- Social Science
- Social Science – Philosophy
- Social Science – Sociology
- Social Sciences
- Social Work
- Society
- Sociology
- some strategies are selected and survive
- someone cannot engage in a Discourse in a less than fully fluent manner. You are either in it or you're not. Discourses are connected with displays of
- Sorting of Boxes According to Height Using PLC Based System
- South University
- South University Online
- Southeastern University
- Southern New Hampshire University
- Southwestern College
- Sp001
- Sports
- Spring 2015 BMGT 1327 CRN 40722)Assignment:Chapter 1 Exam1.award:3 out of3.00 po
- Spring 2015 IS 706 Sec 002: Mid Term Exam IS PROJECT MANAGEMENT Instructio
- St. Petersburg College
- State Center Community College District
- stating that she often told her mother what to do. His responses were unrehearsed and no loose associations in his cognitive processes were observed. Brine was oriented to person
- Statistics
- stock
- Stockbroker Susan Drexler has advised her client as shown in the following table
- Story declared.31 (Links to an external site.)Links to an external site.
- Students, please view the "Submit a Clickable Rubric Assignment" in the Student
- such as can
- such as parental divorce. Peer sociability is strengthened by but also promotes cognitive
- such as reciprocal teaching and cooperative learning. This transforms classrooms into communities of learners and is known as a social-constructivist classroom. Teachers guide the process of learning
- such as semantic bootstrapping (using word meanings to decipher sentence structure) to build their grammar knowledge? Or
- such as those that begin with consonants
- swelling the Confederacy's urban population. At the same time
- SYM-506 | Additional Topics Module 8 DQ 1 A market researcher is interested in k
- SYM-506 | Analysis Using Graphs and Descriptive Statistics Module 2 DQ 1 Referri
- SYM-506 | Discrete and Continuous Probability Distributions Module 4 DQ 1 Provid
- SYM-506 | Hypothesis Testing With Single Samples Module 6 DQ 1 Your mayor just a
- SYM-506 | Hypothesis Testing With Two Samples Module 7 DQ 1 Describe when a z-te
- SYM-506 | Sampling Distribution and Confidence Intervals Module 5 DQ 1 You just
- SYM-506 | Understanding DataModule 1 DQ 1The media often attempts to predict the
- System Engineering
- Take Assessment: Final ExamName Final ExamInstructionsMultiple Attempts This Tes
- Take Assessment: MGT 190 Exam 1 NameMGT 190 Exam 1 Question 1 In most business s
- Tarrant Country College
- Task Name: Phase 1 Individual Project Deliverable Length: 57 slides with speak
- Task Name: Phase 2 Individual Project Deliverable Length: 9001,000 words Det
- Task Name: Phase 3 Individual Project Deliverable Length: 68 slides with speak
- Task Name: Phase 4 Individual Project Deliverable Length: 68 slides with speak
- Task Name: Phase 5 Individual Project Deliverable Length: 810 total slides wit
- TCO 2: In order to address the hospitality labor shortage, employers are:
- TCO 9: Mobil Travel Guides establish ratings for lodging properties by: su
- TCO A: The organization is planning to make a substantial change to the compensa
- Technology
- television
- Tennessee for about twenty years. I was his slave
- Term Paper:A final paper that focuses on the course content, applied in the sett
- terrorism
- TEST BANK Sourcing and Supply Chain Management 4th editionHandfield, Monczka, G
- TEST BANKSourcing and Supply Chain Management 4th editionHandfield, Monczka, Gi
- testing
- Texas Am University
- th[at] if they would lay down their arms and submit to the laws of the United States before the 1st of January
- The ability to perceive and express emotion, assimilate emotion in thought, unde
- the attribute is useful
- the author of the previous selection
- The Capstone Business / Leadership CaseDuring the first class the Professor post
- the Confederacy fought against overwhelming odds; its defeat was inevitable.
- the court also explained that 'no free government now exists in the world
- The directions are attached. However you must read the PDF file first in order to answer the questions.
- the enemy would be in a position to advance upon the line of the Second Corps
- the features that Hal should make available on the site
- The Gamer Company is a video game production company that specializes in educati
- The Global Baby BustScenarioYour RoleKey PlayersAssignmentScenarioMost people t
- The Leadership Project requires each student to lead a group of people (friends
- the long-run aggregate supply curve will:
- the modern Court did not create new law; rather
- the more modernized the society. Modernization therefore has its intellectual foundations in a rationalistic or scientific world view and a commitment to technological development….
- The objective of the Course Project is to give you an opportunity to practice
- the project also serves to establish your literacy in other crucial areas of the security industry
- the project requires 6 inches of pavement thickness if the unit weight of the product is equal to 145 lbs per cubic foot. Using this information
- the psychiatrist stated that Charles was not suicidal and diagnoses him with antisocial personality disorder.(Learning Objectives: 1
- the researchers were interested in how parent and teacher reports of activity compared to actigraph results.
- The sale of government-owned economic resources to private operators is calle
- the traditional classroom and the constructivist classroom. In the traditional classroom the teacher is the sole authority knowledge
- the Union developed supe rior managerial talent to mobilize and organize the North's greater resources for victory in the modern industrialized conflict that the Civil War became.
- The Unit 3 Assignment covers aspects of Units 1, 2 and 3. Respond to the short a
- the various risks to assets; security and safety control operating standards
- The World Bank & IMF in Ghana
- Theatre
- their achievements went beyond what might have been expected. But the South had far too much ground to make up
- then
- Theology
- there is a question as to whether that just crowds out investment in other sectors of the economy. Housing finance while improving access to housing
- thereby
- These are the topics you have to choose from to write the course paper.â¢In tod
- These are the topics you have to choose from to write the course paper.In todayâ
- these findings do not suggest that heavy television viewing causes ADHD. However
- they run the risk of losing their .A) respectB) credibilityC) licenseD) innocenceE) accreditation43) Communicating an idea in such a way that an audience is influenced
- they think about their thinking. This is known as metacognition. When children meet mental challenges
- think about a team you currently work with or have worked with in the past and how well this team has functioned. Think about both the positives and the negatives
- thirty-six percent of the Northern population was already urban as compared to the South's nine and six-tenths percent. As we have already seen
- This assignment has three objectives, to: 1) become familiar with the type and m
- This assignment is designed to show how the economic concept of price discrimina
- This case is based on Chapter 15, Case 15.3 which is found on Page 15-50. Rememb
- this curiosity has led to theories on how language skills develop in childhood.
- THIS PAPER IS LATE_ NEED REALLY GOOD GRADE….Strategic Management: External Ana
- Thomas Nelson Community College
- though almost as legion as Lee's soldiers
- Tim8330
- Title: Successfully identify the mutations of GFPS65T and purification of GFP.
- Tittle: Human Resource Planning and Organizational Strategy In a five-page pape
- to be true of second language acquisition or socially situ ated cognition (Beebe
- to better promote the value and dignity of individuals or groups and to serve others in ways that promote human flourishing.
- to some extent at least
- to submit the following report of op erations of the Armies of the United States
- to usc a Discourse. The most you can do is III It'! them practice being a linguist with you.
- Topic for Discussion:Human resource development, as we discussed, can be a stand
- Topic: The Organizing Process Subject: Management In a five- page paper (exclu
- touching the condition of the colored people in the rebel States.
- Tourism
- Touro College Of Los Angeles
- Training Objectives and SMART Goals PaperBuilding on your assignment from last w
- Translation
- Transportation
- Trident MGt301 full course 2014 latestFUNCTIONS OF MANAGEMENT AND ROLES OF MANAG
- Trident University International
- Troy University
- TRUE or FALSE?1.) The main objective of Risk Management is the mitigation of the
- TRUE/FALSE1. A bottleneck is an operation that has the lowest effective capacity
- True/False1. The order action report indicated which orders are to be released d
- Ty Webb, manager of the Philadelphia Hotel, is considering how to restructure th
- uestion1 Not yet answered Marked out of 20 Flag question Question text Discuss t
- Uncategorized
- Unit 1: Unit 1: Introduction to Human Resource Management – Discussion.next.ecol
- Unit 1: Unit 1: Planning and Organizing a Small Business – Discussion.next.ecoll
- unit 1PART 1: Introduce yourself to your fellow students and instructor. Share
- UNIT 1Question 1: Why is it necessary to strengthen the relationship between bus
- Unit 2 Assignment: General Motors Commitment to DiversityRead the vignette Gener
- Unit 3: Unit 3: Talent Selection and Retention – Discussion.next.ecollege.com/(N
- UNIT 3In what generational cohort do you belongMillennial, Gen X, Baby Boomer,
- Unit 5: Unit 5: Supply Chain Mechanisms – D: Team D Discussion.next.ecollege.com
- UNIT 5Kotler describes the importance of packaging with a series of packaging ob
- UNIT 7 The Marketing Memo on page 496 describes methods that determine how int
- Unit III Case Study Read KFCs Big Game of Chicken, which is a case on pages 260
- Univ of M-Flint MGT 313 Quiz 01 Winter 2015Review Test Submission: Q01User Talal
- University Of California Santa Cruz
- University Of Cumberlands
- University Of Maryland University College
- University of Michigan-Flint MGT 313 Intro to Management Science Winter 2015Revi
- University Of Nevada – Las Vegas
- University Of South Carolina
- University Of South Florida
- University Of The Cumberlands
- University Of Virginia
- University Of West Florida
- University of WindsorCenter for Executive and Professional EducationalMaster of
- Unlv
- unobtrusive
- until the Military power of the rebellion was entirely broken. Believing us to be one people
- upgrade the family cars
- upon the field
- Using the book: Robbins, S.P., & Judge, T.A., 2012, Essentials of Organizational
- validated by the independent expert assurer to ensure that the commercial tension is maintained
- vandalism
- Video Games
- W2 Discussion Due: 07/13/2014 (75-150 words) Should we have ONE BIG UNION? Would
- Wa-Wa
- Walden University
- was the principal force holding the white South together. Without it
- we can always ask about how much ten- 12 """""" or conflict is present between any two of a person's Discourses (Rosaldo
- WEEK 1 .apus.edu/access/content/group/arts-and-humanities-common/Political
- week 1 discussion Baby Boomer Demand (graded Why are the baby boomers so importa
- week 1 Discussion Question Why has there been (and is still) such strong opposit
- WEEK 1 DQ 1Research the definitions of various production and operations managem
- WEEK 1 Exploring the Course Themes (graded) The four course themes of e
- week 1 Forums Question: For the Forums discussion this week: after reading th
- WEEK 1 Module 1 Discussion Do you believe that corporations should b
- WEEK 1 Striking a Balance (graded) When efficiency, equity, voice, and
- WEEK 1 Why and how has human resources changed due to the technology revolution
- week 1Assignment To complete the following assignment, go to this week's Assignm
- WEEK 1Case Study: Keflavik Paper Company (graded)Read Case Study 3.1The Keflavik
- week 1Discussions To participate in the following discussions, go to this week's
- week 1Take some time to discuss, in your opinion, the most interesting material
- WEEK 1The Purpose of HRM (graded) Human Resources Management (HRM)what a mouthfu
- Week 2 Assignment Productivity Problem SetDetails:Complete Objective Questions 1
- Week 2 AssignmentProductivity Problem Set Details: Complete Objective Question
- Week 2 Chapte3 Cost-Volume Relationship Managerial Accounting for Managers 3rd E
- Week 2 Social Media Policies: Are They Legal? .equella.ecollege.com/file/f50b
- WEEK 2Project Scope (graded) As we have seen this week, project scope creation i
- Week 3 Assignment Project Management Problem Set Details: Complete Objective Q
- week 3 Discussion Question Why are employers less likely to approve coa
- WEEK 3 Healthcare Cost Management(graded) Many Americans benefit from t
- WEEK 3 HR Strategy and the Employee Voice (graded) Human resource profe
- Week 3 O*NET Assignment .equella.ecollege.com/file/c7bbe571-8052-434e-a412-7f
- WEEK 3 Project Schedule (graded) To develop a schedule for a project, w
- week 3 Recruitment is not the only activity that affects an organization's abi
- WEEK 3Interviewing (graded) Interviewing methods are extremely varied so this th
- week 3Module 3 Discussion Question 1 Research the World Bank at www.worldbank.or
- WEEK 3Project Schedule (graded)To develop a schedule for a project, we will use
- WEEK 3Topic for Discussion:Most of us have either given or participated in lectu
- Week 4 AssignmentCapacity Planning Problem Set Details: Complete Objective Q
- WEEK 4Risk Management (graded) Assume you have just been assigned to a project r
- Week 5 AssignmentProcess Analysis Problem Set Details: Complete Objective Que
- WEEK 5 Interest Arbitration (graded) List the pros and cons of interest
- week 5 Let's consider the different methods of assessment and how they are use
- WEEK 5 Performance Appraisals (graded) What are the different performan
- WEEK 5Case Study: The Problems of Multitasking (graded) Read Case Study 12.1: Th
- WEEK 5Case Study: The Problems of Multitasking (graded)Read Case Study 12.1: The
- WEEK 5In your opinion, based on the twelve guidelines listed in our reading ma
- WEEK 5Rules and Regulations (graded) Discuss some of the legislation that helps
- WEEK 5Topic for Discussion:Think about the last time you had a problem or were d
- Week 6 AssignmentLayout Design Problem Set Details: Complete Objective Questio
- WEEK 6Project Communications (graded) Discuss the importance of communication an
- Week 7 AssignmentQuality Management Problem Set Details: Complete Objective Qu
- WEEK 7 Benefits Communication (graded) Does your current (or previous)
- week 7 Employment-at-will involves the right of either the employer or employe
- WEEK 7 Workforce Change (graded) What recommendations would you make to
- WEEK 7Employee Engagement (graded) Employee engagement has become pop
- week 7Module 7 Discussion Question 1 Visit the domestic and international web si
- week 7Project Baseline (graded) We have now moved from the planning stage to the
- WEEK 7Topic for Discussion:Please discuss the main functions of organizational d
- week 8 : Final Exam – Final ExamPage 1Question 1. 1. (TCO 1) Explain why the tr
- Week 8 AssignmentInventory Management Problem Set Details: Complete Objective
- Week 8 AssignmentSupply Management Problem Set Week 8 AssignmentSupply Managemen
- Week Three Homework Assignment – Linear Regression The personnel director for
- Week Three Homework Assignment – Linear RegressionThe personnel director for a l
- WEEK1 Benefit Plans (graded) Employer-sponsored benefits reflect the culture and
- WEEK3What are the important considerations when planning and setting up a new
- Weems
- were no more able to rise above narrow and selfish concerns than other segments of southern soci ety. Because of their influence
- West Valley College
- West Virginia University
- Western New England University
- What is the key concept of Sandmans High Hazard/High Outrage type of risk commun
- what role does slavery play in his analysis?
- when Generals Hancock and Gibbon rode along the lines of their troops; and at once cheer after cheer-not rebel mongrel cries
- where the requirements for APA Style are specified."
- whether good or bad. The intent of the short research projects is to dig a little deeper into some of the topics
- which appeared imminent before each of the next three turning points.
- which eventually leads to the ability to organize speech into the phonemic categories of their own language. Older infants begin to detect the internal structure of sentences and words
- which follow a subject-verb-object order. Once this occurs
- which measures impulsivity
- which measures participants' ability to inhibit automatic responses
- Which one of the following is considered to be a nonmarket stakeholder of bus
- which triggers the vulnerability. As soon as the user downloads this shortcut file on Windows 10; windows explorer will
- will they have the authority to make changes as necessary?Are the individuals with the right skills in place to implement these changes? If not
- Winter 2015FinalBasics1. (4 points) Match each description to the project manage
- with a population hostile to the government
- working together
- worship
- would be Japan
- Write a 350- to 700-word paperthat defines and compares and contrasts the follow
- Write a 700- to 900-word paper in which you do the following: Explain the role o
- Write a paper in which you consider the .ncu.edu/homework/details?assignmentid=1
- Write your mock Concept Paper using the Concept Paper template found in the Diss
- Writing
- written and oral communication skills
- Written Assignment 2InstructionsInstructions: You have been hired by the XYZ Com
- wrote a letter to ą friend in 11 Baltimore after Robert E. Lee's army entered Frederick
- Ych635 Psychology Of Learning
- you believe you can provide the CIO with the information he needs.
- you could have witnessed the transit of the Rebel army through our streets [of Frederick
- You Decide Scenario Summary Your Role/Assignment Key Players Scenario Summary C
- you have gained in this course relate to the following professional occupational positions:
- you have to go through the process of retrieval. Retrieval of information from our long-term knowledge base occurs in three ways: recognition
- you may group related items (e.g. all dairy products together)
- you need to put 2 vertical and 2 horizontal lines evenly spaced to make 9 spaces of the same length and height. You need to them line up the subject of the shot with one of the 4 lines
- you will learn how to search for scholarly
- you will review the Procon.org Website in order to gather information. Then
- Young People
Recent Comments